Natural Cooling
Passive cooling techniques can be used to reduce, and in some cases eliminate, mechanical air conditioning requirements in areas where cooling is a dominant problem. The cost and energy effectiveness of these options are both worth considering by homeowner and builders. Contained within this section are rules of thumb and an explanation or the essentials of passive cooling systems.
In many parts of the southwest, summer cooling is as important as winter heating. In the arid part of the country, cooling is the primary design consideration.
Thermal comfort in summer means more than keeping the indoor air temperature below 75°. High temperatures, or high humidity (or both) can lead to excessive discomfort. Fortunately, the regions of high summer temperatures are quite arid (relative humidity is usually low). The only regions of fairly high humidity, the coastal regions, are also among the coolest parts of the region in summer.
There are three major sources of unwanted summer heat: direct solar impacts on a building and through windows and skylights; heat transfer and infiltration, of exterior high temperatures, through the materials and elements of the structure; and the internal heat produced by appliances, equipment, and inhabitants. Of the three, the first is potentially the greatest problem in the southwest, but it is usually the easiest to control. Table 14 lists approximate heat gains from each source for typical single-family detached homes in a climate where the temperature averages 75° F on a July day. The homes are built to local energy codes and are oriented east-west, and have two-thirds of the total glazing facing south. The remaining glass is located on the east and west walls, and all glass is completely unshaded. Even assuming that sunlight could be excluded from the interior in summer (a difficult feat), these homes would experience excess heat loads of 250 to 450 thousand BTU per day in July. Worse yet, the houses would require about 4-8 tons of air conditioning each to handle peak heat gains and keep the rooms comfortable in the afternoon.
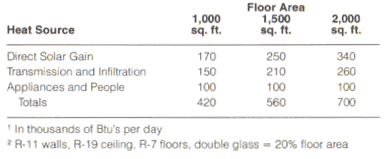
Table 14. July heat gains1 in typical tract homes2.
HEAT GAIN CONTROL
Many of the principles and techniques of passive solar heating are adaptable to natural cooling. Insulation and weather-stripping that prevent heat loss in the winter will also retard heat gain during summer. Movable insulating shutters for winter nighttime containment of heat gain can also be used to reduce summertime daytime heat gains. Inside the house, thermal mass such as masonry walls and floors, act as "heat sponges", absorbing heat and slowing internal temperature rise on hot days, and can be cooled down by nighttime ventilating (at the beginning and end of the summer season) and by use mechanical cooling during off-peak cost hours (nighttime). Suitably placed near a window, skylight, or vent, the same thermal mass can be exposed to cool night air to release the heat absorbed from the space earlier in the day. Finally, earth integrated buildings, embedded into the ground, benefit from the lower difference between interior and exterior surface temperature.
For optimum summer cooling, a building's surroundings should be designed to minimize summer sunlight striking external surfaces, and to prevent surrounding area heat re-radiation and reflection. Great temperature differentials between desert exterior conditions of 110+ degrees and 78 degrees required for interior comfort can be tempered using "thermal decompression" zones that become increasingly more effective as one nears the building. Mitigation of undesirable summer direct sun and thermal impacts is achieved through use of vegetation i.e. deciduous trees which interrupt the summer sun's direct path, and ground covers which prevent ground reflection as well as keep the earth's surface cooler thereby preventing re-radiation. One moves out of intense direct sun and heat through vegetation that filters sunlight and shades the ground; then through a more densely filtered zone with ground covers; then through a patio area with vegetation, trellises and water features; into a tempered building entry ("thermal lock"); and finally into the building proper. This movement, 110 degrees stepping down in stages to 78 degrees, allows the body to adjust properly, and provides the best means of arriving at a lesser differentiation between the building's perimeter wall interior and exterior surface temperatures. It is this difference, between interior and exterior surface temperature, that exacerbates the amount and rate of heat flow through the material. Glazing should be minimized on the roof and the east and west walls where summer sunlight is most intense.
Intense direct solar impacts from the sun rising in the east are equal to those of the setting west sun. The reason we feel the setting sun impact more is due to the added thermal impact of the earth reradiating the heat it has gained during the day. The summer sun is much higher in the sky and has a negative impact on skylights and roof windows and lead to enormous solar heat gains. They should not be used in hot climates unless they are insulated and/or shaded. Vertical south facing glass (windows, clerestories, etc.) with overhangs or shades, present fewer problems but are still adversely affected by exterior air temperature. A horizontal overhang or an awning above a south window is an inexpensive, effective solution. If it protrudes to half the window height (Fig. 21), such an overhang will shade the window completely from early May to mid-August, yet allow for winter sun access. A trellis with deciduous vines can be used. Another good strategy is the use of deciduous trees that shade the south face and roof during the summer. All these shading methods work equally well with Trombe walls, water walls, greenhouses, and other south-wall passive solar collector strategies.
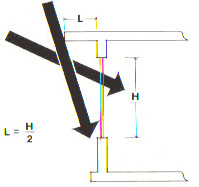
Figure 21. Shading devices should be sized using the above graphic method.
Mitigation for the roof and the east and west walls requires a different approach. Since the sun is low in the horizon during sunrise and sunset, overhangs are not effective for solar mitigation and vertical shading is in order. Vegetation is perhaps the most effective way of keeping the intense morning and afternoon sun off the east and west walls and windows, but care must be taken to avoid blockage of nighttime summer breezes that can be part of the diurnal cooling strategy. If vegetation is impractical, a combination of tinted or reflecting glass and exterior shades or shade screens that roll down over east and west windows are an effective strategy. Additionally, light-colored paints and materials on the roof and the walls are effective in reflecting away most of the sunlight that makes it past your shading.
CONVECTIVE COOLING MODELS
The heat gain control methods discussed above should suffice to keep room temperatures comfortable in houses built where mild summer temperatures are the rule. But there are many other regions of the southwest, particularly the desert areas, where additional cooling will usually be necessary. The next step in natural cooling is to take advantage of "convective" cooling methods - those which use the prevailing winds and natural, gravity-induced convection to ventilate a house at the appropriate times of the day.
The oldest, straightforward convective method admits cool night air to drive out the warm air. If breezes are predominant, high vents or open windows on the leeward side (away from prevailing breeze) will let the hottest air, located near the ceiling, escape. The cooler night air sweeping in through low open vents or windows on the windward side will replace this hot air and bring relief. To get the best cooling rates, leeward openings should have substantially larger total area (50% to 100% larger) than those on the windward side of the house (Fig. 22).
If there are only light breezes at the site, natural convection can still be used to ventilate and cool a house as long as the outdoor air is cooler than the indoor air at the peak of the house. Since warm air rises, vents located at high points in the interior will allow warm air to escape while cooler outdoor air flows in through low vents to replace it (Fig. 23). The coolest air around a house is usually found on the north side, especially if this area is well shaded by trees or shrubs and has water features. Cool air intake vents are best located as low as possible on the north side. The greater the height difference between the low and high vents, the faster the flow of natural convection and the more heat mitigation can occur.
![]() |
![]() |
Figure 22. Locate cool air inlet vent on the building side which receives predominant cool summer breezes. | Figure 23. To aid in natural ventilation, during summer use high ceiling vaults, and thermal chimneys to promote rapid air changes. |
There are two basic ways to enhance the convective cooling rate: 1) increase the volume of air escaping per minute, or 2) bring in cooler air. If Delta T is the temperature difference between exiting indoor air and incoming outdoor air, the overall cooling rate in BTU's per hour is given by the simple equation:
Cooling rate = 1.08 x V x DT
where V is the volume of air escaping in cubic feet per minute. Table 15 contains sample values of the cooling rate for selected air flow rates and temperature differences. For example, an air flow velocity of 1-2 feet/sec. through a vent of 10 square feet will result in air flow rates between 500 and 1000 cubic feet per minute. If incoming air is 10 degrees cooler than the indoor air, the overall cooling rate will be about 5 to 10 thousand BTU's per hour.
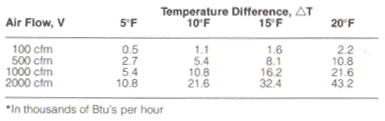
Table 15. Convective cooling rates.
To a point, increasing the vent area will increase the airflow rate by natural convection. Turbine vents at the roof peak are one way to enhance airflow and improve the cooling rate. Even gentle breezes flowing up and over the roof peak create an upward suction that draws out warm interior air (Fig. 24). An even better approach is to use solar radiation to induce a more rapid flow. One of the many possible approaches, shown in Figure 25, uses a Trombe wall vented to the outside. Sunlight striking the concrete wall will heat the air in the space between glass and wall to temperatures above 150°F. This very hot air rises quickly and escapes, drawing cool air into the house through low vents on the north wall. Additionally, specifically constructed "solar chimneys", composed of passive air heaters with seasonal dampers can be incorporated where solar heated air can be dumped into the building in the winter, and used as a "ventilator driver" in the summer to draw outdoor air through a house and ventilate it. Frequently, they can induce air velocities of 1-2 feet per second.
Another convective cooling strategy is the drawing of outdoor air is drawn through tubes buried in the ground and dumped into the house. Made of material that allows easy thermal transfer, these tubes are buried several feet deep to avoid the warmer daytime surface temperatures. Warm outdoor air entering the tube gives up its heat to the cooler earth, and cools substantially before entering the house (Fig. 26). Thermal saturation of the surrounding earth must be addressed, by means of surface landscaping and watering, thereby removing the gained thermal energy from the tube/earth transfers. Though condensation is rarely a problem in dry climates, such tubes should be sloped slightly and have adequate drainage to insure that water build-up doesn't block the passage of air. The intake end should be screened and placed in a shady spot away from foot traffic. When properly built and sized, these underground tubes can supply cool air during the peak load daytime even in the hottest climates.
![]() |
![]() |
|
Figure 24. Wind turbines can be used to increase the ventilation rate of rooms. | Figure 25. An indirect gain mass wall can be used to significantly increase ventilation rates in adjoining spaces. | |
![]() |
||
Figure 26. By using undergrade air chambers, significant sensible cooling can be obtained. |
RADIATIVE COOLING METHODS
The exterior water wall and roof pond systems mentioned earlier are also very effective summer cooling strategies. In the cooling cycle (Fig. 27), insulating panels remain closed by day to reject unwanted solar heat. The cool ponds act as "thermal sponges", absorbing room heat conducted through the interior ceiling ceiling (metal deck) supporting them. At night. panels are rolled back, exposing the ponds to the black body of the night sky and to the cooler night air and breeze. The ponds lose heat by radiation to the night sky and by natural convection to the air. Roof pond systems are particularly effective in regions of low humidity and clear summer nights. The conditions exist in most of the southern tier, where the cooling demand is greatest. If conditions are less than ideal, augmented heat dissipation by evaporation can be integrated (see following section).
For best cooling results, ponds can range from 6-12 inches deep, depending on location and local conditions, and should cover as much of the roof as possible. An average tract home in the southwest, with good heat gain control, can easily gain 200,000 to 400,000 BTU's on a hot July day. A 6 inch deep roof pond covering the entire roof, will rise in temperature by only 4-8°F from this heat gain, and with nighttime cooling rates of 25-30 BTU/hr/ft' (Fig. 27), all this excess heat can be released to the outside by daybreak.
A few considerations for roof systems and ponds. They should have an unobstructed path toward the zenith (directly overhead). Adjacent trees, walls and other buildings can impact the cooling rate by reducing radiation to the night sky. Trees and walls also absorb solar heat by day and radiate this energy into the ponds at night. Cloud cover can interfere with the cooling performance of a roof pond system. For this reason, roof ponds are less effective in coastal areas where a low cloud layer or dense fog frequently rolls in off the ocean in the evening. Fortunately, however, such coastal areas need little if any cooling during the summer.
![]() |
Figure 27. Roof bonds utilizing cool, clear nightskys can provide total cooling in many sections of the state. TOP - panels are kept closed during the day. BOTTOM - panels are opened after dusk to radiate out the absorbed day time interior heat. |
EVAPORATIVE COOLING METHODS
When water evaporates it absorbs a large amount of heat from its surroundings (about 1000 BTU per pound of water evaporated). The most familiar example of this is the cooling effect of evaporating perspiration on the human skin. In arid, hot climates body temperature is partially controlled by the rapid evaporation of perspiration from the surface of the skin. In hot climates with high atmospheric moisture the cooling effect is less because the high moisture content of the surrounding air. In both situations, however, the evaporation rate is raised as air movement is increased. Both of these facts can be applied to natural cooling of structures.
Evaporative methods can be used to enhance the cooling rates in convective cooling systems. One way of doing this is to bring the outdoor air into the house through a moist filter or pad as shown in Figure 28. The familiar evaporative cooler, precursor to the air conditioner, is a mechanical system which uses these principles with a motor to force air movement and distribution. Passive cooling strategies with earth tubes and/or cool towers use the same principles but utilize natural systems for air drivers and distribution. If underground intake pipes are made from a porous material, and ground above them is well cool and watered, some evaporation will occur at the inner surface of the pipe.
![]() |
Figure 28. Swamp cooler - drier outside air is drawn through a moist pad. As moisture is picked up by the air heat is absorbed by the water, the result is cooler air. |
Cool towers utilize wet cooling pads, and the force of gravity. Heavier, cooled air "falls", via gravity, into the building and its momentum floods the habitable area. This cool tower action, as well as that of the earth cooling tubes, can be enhanced and distribution extended, by the placement of thermal chimney "drivers" which can pull the cooled air through the building with an increase in both air quantity and velocity. In either case, the cooler air now has a higher relative humidity, but this is not usually a problem and can even be a benefit in arid climates.
In some areas, there may be a time of higher humidity (desert monsoon season). While sensible heat continues to be mitigated by passive cooling techniques, the latent heat contained in the humid air is more difficult to dissipate, which renders evaporative cooling less effective. The integration of a air dehumidification system easily corrects this short term problematic condition.
Evaporative cooling strategies are well suited to those areas of the southwest with the most severe cooling requirements. In the desert areas of the South, the warm night air (80 degrees+) may impede natural convection heat dissipation from a roof pond cooling system. That is one of the reasons why the cooling rate falls to about 25 BTU/hr/ft^2 in the extreme southeast corner of the state (Fig. 29). Simple introduction of a thin water layer over the water containment surface can increase the overall cooling rate of the roof by 50-100 percent due to the resulting evaporation.
In the most severe climates where nighttime air temperatures often remain above 90°F in summer, sprays can be used to achieve maximum natural cooling, at standard roofs and roof cooling systems like the roof pond strategy. In the summer, sprays can be used to achieve optimum natural cooling. In the approach shown in Figure 30, water is pumped to sprinklers along the peak of a house and allowed to trickle down a sloping roof. The rate of evaporation is greatly enhanced in such a system because a much larger surface area is exposed to the night air. Roof sprays rely on a little external power to get the water to the roof and hence do not qualify as completely passive systems. But the total amount of energy consumed for pumping is very minimal compared to the energy saved by the added cooling rate attained. Excess water can be captured and reused or used elsewhere on the site.
![]()
|
![]()
|
Figure 29. Roof top sprinkler - combined radiative and evaporative cooling can be integrated together to increase the rate of cooling | Figure 30. Open pond with water wall - combined systems can be devised to provide direct cooling. For all interior spaces. |
A passive evaporative system developed in California is shown in Fig. 31. An open pool of water located above the living spaces on the north side of a house is shaded from the summer sun but exposed to the cool north sky both day and night. Evaporation from the pool surface, aided by radiation and natural convection, keeps the water in this pool 30°F below the outside air temperature on a hot summer day, without the use of movable insulating panels. Natural convection brings this cool water into the house and draws heat back up to the pool as shown.
With all evaporative cooling methods, it is important to maximize airflow across the exposed water. Fresh air must be continually available to replace the humid air being built up near or over the water. Failing this, air will be quickly saturated with water vapor, and the evaporation and cooling rates will decline abruptly. Lips, edges and other structures or buildings that could block or deflect prevailing winds away from the water surfaces should be studiously avoided. Sometimes, a small fan to disturb the air over a pond will greatly aid the evaporation rate on a hot, sultry day or night.
Even with direct, active evaporative cooler systems, provision of interior thermal mass combined with direct evaporative cooling is a combination that works effectively. During the day, the structure can utilize the stored coolth in the walls and floors, and maintain an improved level of comfort while reducing power requirements of direct evaporative cooler system. In many areas of the southwest which are considered hot, arid zones, periods of higher humidity renders mechanical evaporative cooling unsatisfactory even when optimized techniques are used. A solution to this is the two-stage evaporative cooling system, which has been shown to be an effective alternative to direct evaporative cooling or refrigerated air-conditioning.
While not a passive system, two-stage evaporative cooling is an important element to be considered as part of passive cooling strategies. Cooling is accomplished by pre-cooling ambient air without humidification before further cooling by evaporation. The cool air entering the structure is then exhausted, typically through areas of heat gain such as windows or the attic. The pre-cooling may be accomplished by a combined cooling tower, heat exchanger unit, or by nocturnally cooled rock bed through which air is drawn. The second stage, evaporative cooling, is accomplished by a standard commercial evaporative cooling device, or by passive cooling elements of earth tubes or cool towers. Rock bed mechanical cooling has been used extensively in Australia with high degrees of effectiveness.
Two-stage evaporative system can also be combined with active and hybrid solar heating systems using the same storage (rock bed) system for both seasons. Working systems have been developed and demonstrated. This type of system is necessarily suited for new construction because of the requirement for the rock bed, which is most effectively located beneath the structure. It works well during hot, humid periods in the southwest using only slightly more power than direct evaporative cooling and the comfort attained is similar to that of refrigerated air-conditioning.
A typical system consisting of two evaporative coolers and a large rock bed is shown in Figure 31. At night, one evaporative cooler cools the rock bed while the other cools the house using a one-stage evaporative cooler. During the day, hot outside air is drawn through the night-cooled rock bed where it is pre-cooled before entering the main house evaporative cooler. Since no moisture has been deposited in the rock bed, the pre-cooled air has not had moisture introduced into the house. An attractive feature of this type of system is the combining of heating and cooling systems in order to make the best possible use of components during the entire year. An air heater may be used to provide hot air during the heating season to the rock bed where the rock bed, fans, ducts and many of the control systems are used both during the heating and cooling season.
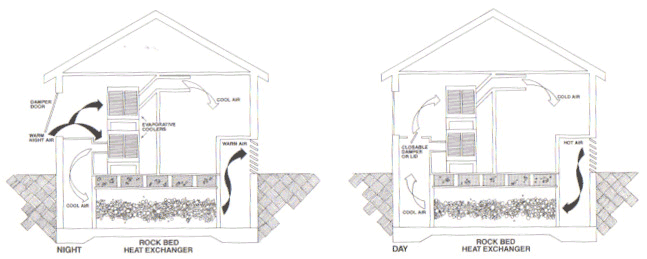
Figure 31. Schematic diagram of two stage evaporative cooling with rockbed.
Recuperative and regenerative evaporative cooling options are other methods to produce greater comfort using evaporative cooling. These techniques use the relatively cool air exhausted from the structure to improve the performance of the evaporative cooling device. Evaporatively cooled water reduces in temperature the ambient air in the heat exchanger without humidification as it enters the structure. The cool, dry air warms a few degrees as it passes through the structure and exits through the evaporative cooling device or a cooling structure. Since the exiting air is cool and dry, the wet bulb temperature is lower and the water produced by the evaporative cooling device is cooler than if ambient air were used. The rock bed heat exchanger and the evaporative cooling device could be combined into a single unit. If the rock bed is used to store heat in the winter, the cost effectiveness of the system is improved.
NOTE: The psychometric chart should be used at all times to analyze the effect of changing air conditions in these systems. As a rule of thumb, pre-cooling the air ten degrees will cause a three degree decrease in the output temperatures of an evaporative air cooler. The improper use of this rule can lead to errors of judgment when analyzing the results of changing conditions.
Because of the large volumes of air that are moved in an effective evaporative system, the ducts must be large and appropriately sized. Typically, evaporative cooler ducts are at least three times the cross-section area of ducts refrigeration; ducts should be laid out using the shortest route possible and a minimum of turns. Evaporative cooling has been shown to be an effective alternative to refrigerated air-conditioning throughout the desert regions of the southwest. The selection of the particular evaporative cooling techniques must be made carefully through analyzing the local climatic conditions. These cooling systems should be integrated into the design of the home and where possible, with the design of the solar heating system. By integrating these systems at the design stage, greater efficiencies and more attractive economics can be obtained.
- Introduction to Solar Energy
- Passive Solar Architecture - Heating
- Natural Cooling
- Glossary